Bioprinting Pancreatic Organoids
At IMOS, pancreatic cancer patient-derived organoids (PDOs) as well as pluripotent stem cell (PSC)-derived pancreatic acinar- and duct-like organoids (PALOs/PDLOs) have emerged as a robust preclinical model. IMOS scientists have demonstrated that these organoid models faithfully recapitulate the unique histoarchitecture, function, phenotypic and genotypic profiles, and heterogeneity of pancreatic healthy tissue and tumors. Additionally, PDOs effectively mimic cancer-intrinsic vulnerabilities, rendering them an ideal platform for patient therapeutic profiling and drug screening approaches. The bioengineering group at IMOS is dedicated to advancing the field of cancer research through the application of cutting-edge bioprinting technology. Specifically, we are focusing our efforts on pancreatic organoid and developmentally-relevant as well as cancerous niche printing in precise geometrical composition, utilizing the capabilities of the BioAssemblyBot 400 to create intricate, functional structures with a range of different cell types, densities, and printable biomaterials.
The BioAssemblyBot 400 represents a state-of-the-art technology that has been designed specifically to automate the biofabrication process. Leveraging a suite of advanced bioprinting techniques, this system can generate three-dimensional living tissues, organs, and even complete organisms. The machine's ability to deposit multiple bioinks with a high degree of precision, resolution (approx. 100µm), and accuracy enables the creation of complex structures composed of diverse cell types and biomaterials. This cutting-edge device has the potential to fundamentally transform the field of cancer research but also regenerative medicine by providing a dependable and efficient approach for generating functional multicellular tissues. Furthermore, its high-throughput capabilities render it a highly appealing option for a range of applications including drug discovery and toxicity testing.
Our team is exploring various printing patterns and configurations, with the aim of contributing to the development of innovative treatment options for pancreatic diseases, as well as to advance our understanding of the complex processes involved in tissue development and carcinogenesis. We are also investigating novel bioinks, incorporating, e.g., growth factors, peptides and signaling molecules to enhance the functionality of the printed organoids.
Research topics
As part of our effort to advance pancreatic differentiation protocols, one of the main projects in our group involves printing differentiating PDLOs derived from an established differentiation protocol in our lab. Our goal is to create highly organized structures that accurately mimic the 3D architecture of pancreatic ducts to gain further insights into branching morphogenesis-related biology. To achieve this, we are utilizing a branching design, which involves printing pancreatic organoids in a specific pattern to form interconnected PDLO-channels that resemble the natural structure of pancreatic ducts including main duct components and small ducts.
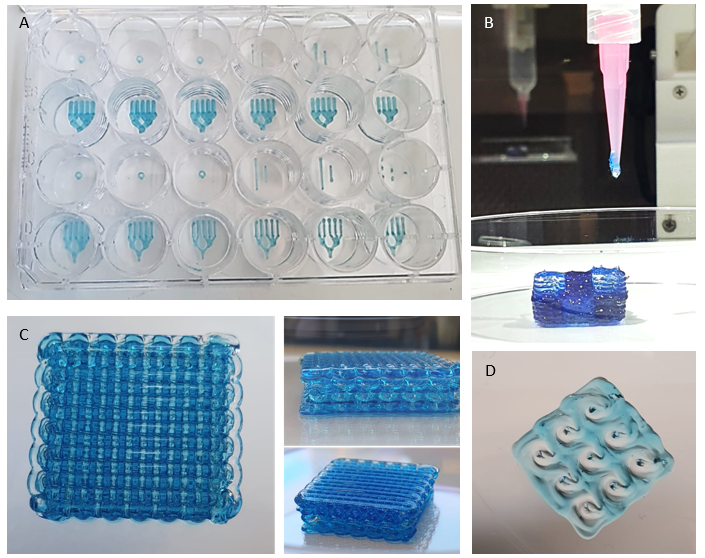
To advance co-culture models, our group is also investigating the potential of co-culturing different cell types with organoids in a geometrically pre-set condition. Specifically, we focus on the interaction of PDOs with stromal compartments, consisting mainly of cancer-associated fibroblasts but also immune, endothelial, and neuronal cells. In particular, we study the instruction and phenotypic alterations of stromal cells through inductive cues from the organoids. This approach could result in the development of more physiologically relevant models of pancreatic cancer, allowing for better insights into the underlying mechanisms of tumor-stroma interaction and the discovery of new therapeutic targets.
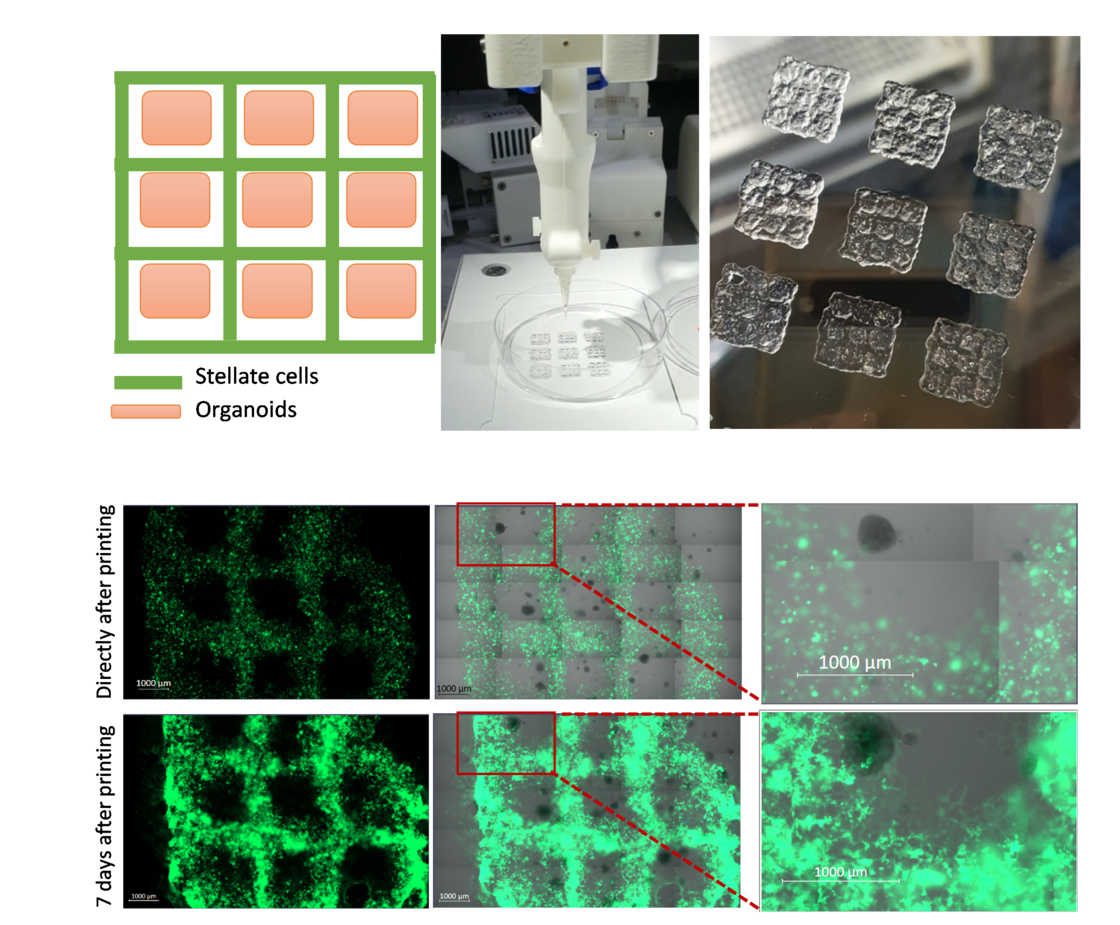
The scanning capability of the BioAssemblyBot 400 not only allows for precise deposition of cells and bioinks in various well plate and petri dish formats, but also enables printing on a variety of also irregular surfaces. Currently, we are applying this set-up to combine the “scan-and-print” capability with other complex models such as our porcine urinary bladder (PUB) organ culture- or the chorio-allantoic membrane (CAM)-model to further add additional but also highly relevant layers of complexity to our models.
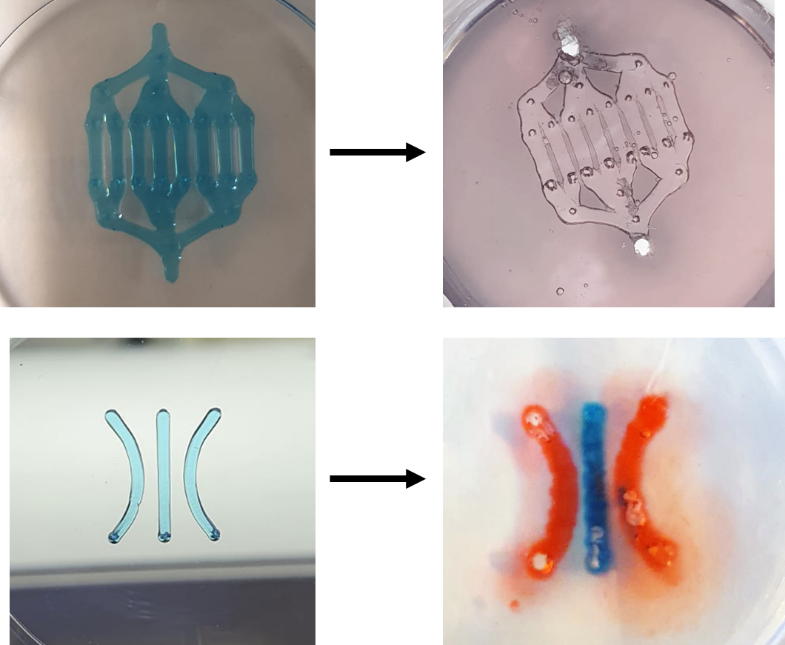
One specific technique that can be used to create microfluidic chips with bioprinting is the addition of sacrificial channels. In this approach, temporary channels made of a removable material are first printed onto the substrate along with the rest of the structures. Once the permanent structures are printed, the sacrificial channels are removed, leaving behind a microfluidic network. We are currently working on this technique to provide microfluidic chips with greater control over channel dimensions and orientation, as well as the potential for creating enclosed microenvironments. These microfluidic chips can be used for applications such as drug screening, cell culture, and organ-on-a-chip devices.